Thesis subject
Summary PhD thesis Wouter van Veen: Aerodynamics of Insect Flight
Insects are flying all around us. However, answering the question: How do insects fly? is difficult. In this thesis, we set out to uncover the relation between the movement of the wing of an insect and the aerodynamic forces. For this we used two Dipteran species as model organisms for low frequency high amplitude and high frequency low amplitude fliers.
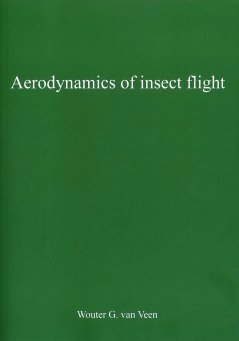
In chapter 2, we revisited the two aerodynamic mechanisms based on the pitch rate motion of the wing: the stroke pitch interactions and the pitch rate forces. We used a large parametric study based on computational fluid dynamics to uncover the relation between the kinematics and the aerodynamic forces. The aerodynamic forces as a result of the pitch motion with constant angular rate broke up into two components. The first component is only dependent on the pitch rate of the wing. The second component is an interaction component between the stroke rate and the pitch rate. To assess the influence of the wing morphology we included seven additional wing morphologies. The inclusion of these additional wing morphologies showed that the stroke interaction forces are related to both the spanwise and chordwise second moment of area. Furthermore, the pitch rate forces where dependent on the offset between the pitch axis and the symmetry axis of the wing.
We used a similar approach for chapter 3 as we used in chapter 2. With this parametric study we set out to uncover the relation between the aerodynamic forces and the stroke motion of the wing for a nonconstant stroke rate. We designed a set of wing kinematics such that the aerodynamic forces due to the stroke rate, stroke acceleration and the interaction between the two, could be found. We included an additional set of wing morphologies to explicitly test the dependence of these forces on the wing morphology. The stroke acceleration forces depend on a scaling parameter that scales with the chord length and the length of the wing. At the end of this chapter we used a quasisteady model to apply our model to the kinematics of the fruit fly and the mosquito. With the use of this model we were able to show that the stroke acceleration forces become more prominent for highfrequency, lowamplitude flappers, such as mosquitoes.
In chapter 4, we took another look at the pitch motion of the wing. Unlike we did in chapter 2, we did not use a constant pitch rate. We again used a systematic parametric study based on computational fluid dynamics to uncover the relation between the stroke rate forces, pitch rate forces, pitch acceleration forces, and the interactions between each component. Here, we tested the validity of the twodimensional model often used to model the pitch acceleration forces in flapping flight. Furthermore, we included a set of four different kinematic cases, which enabled us to explicitly test the dependence of the forces on the direction of motion of the wing. From this we learned that the forces generated by an accelerating pitch up wing cannot be simply translated into a model for an accelerating pitch down wing. With the inclusion of several additional wing morphologies we showed that the offset between the pitch axis and the symmetry axis is not only important for the pitch rate forces, but also for the pitch acceleration forces.
In chapter 5, we used the takeoff of the mosquito to test the influence of the substrate on the generation of aerodynamic forces. We simulated several lean and blood fed mosquitoes taking off from a substrate using computational fluid dynamics. From these simulations we showed that the ground did not have any effect on the aerodynamic forces acting on the wings of the animal. However, the substrate did have an important effect on the generation of the forces of the insect. The mosquitoes used a combination of leg pushoff forces and aerodynamic forces to takeoff. Furthermore, the insect used its legs to generate pitch up torques during takeoff.
In chapter 6, we gathered the knowledge gained in the previous chapters and build a quasisteady model. In addition, we simulated the aerodynamic forces of a hovering fruit fly and mosquito, which gave us a comparison for the quasisteady model. From this comparison, we learned that the quasisteady model based on the aerodynamic mechanisms found in this thesis led to a poor predictive model. However, the model did uncover that mosquitoes rely more other aerodynamic mechanisms apart from the stroke rate forces. At the end of chapter 6, we applied the quasisteady model to the takeoff kinematics we used in chapter 5. This time the quasisteady model showed even a bigger difference, mainly overpredicting the aerodynamic forces. This led us to hypothesize that the body motion of the insect also has a large interaction with the aerodynamic forces. Lastly, we used the kinematics of a lean mosquito and gradually changed these kinematics to that of a fed mosquito. Here, we showed that the lift is altered through a change in mainly the stroke rate forces.