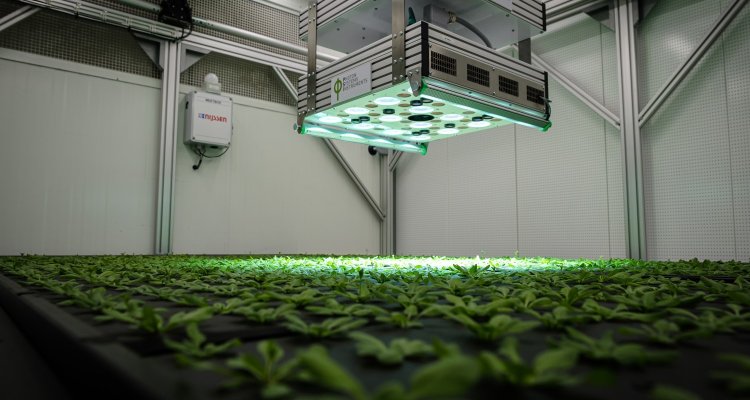
Project
Improving photosynthesis by understanding cyto-nuclear interactions
Proteins whose coordinated action mediate photosynthesis are both nuclear and organellar encoded. Variation for nuclear and organellar genes results in cyto-nuclear interactions. Unravelling the genetic variation for these cyto-nuclear interactions is difficult due to the different modes of inheritance between the nuclear and organellar genomes. Within this project a novel method to generate new combinations between organellar and nuclear genomes in A. thaliana is developed. Using this method the cyto-nuclear interaction are disentangled to reveal the genetic architecture underlying photosynthetic variation.
Background
Improving photosynthetic performance has been identified as the last unimproved component of crop yield. Comparing photosynthesis between genotypes within one species shows there is ample genetic variation for photosynthesis. This genetic variation may be used to improve photosynthesis. Yet, plant breeding has only marginally improved photosynthesis via selection on yield due to the complex genetic architecture underlying photosynthetic variation. Exemplifying this, the proteins whose coordinated action mediate photosynthesis are both nuclear and organellar encoded. Variation for nuclear and organellar genes results in cyto-nuclear interactions. Unravelling these cyto-nuclear interactions can help to develop approaches to incorporate the genetic variation for photosynthesis in breeding programs.
Project description
Organellar genomes (‘plasmotypes’) are inherited maternally, unlike nuclear genomes (‘nucleotypes’), that are inherited from both parents. Consequently, studying the plasmotype variation via conventional mapping populations is significantly more difficult than nucleotype variation. As one approach to disentangle the cyto-nuclear interactions, genotypes with new combinations of nucleotypes and plasmotypes can be made. The genotypes with de novo nucleotype-plasmotype combinations are referred to as cybrids. Traditionally, cybrid production relied on recurrent backcrossing, but this can be a lengthy process and residual nuclear introgressions can continue to influence phenotypic variation. In this project we developed a new method that enables the complete replacement of the nuclear genome within one generation, using a maternal haploid inducer line in Arabidopsis thaliana. This method has been used to unravel the cyto-nuclear interactions for photosynthetic variation.
Results
An initial cybrid panel was constructed covering all combinations between the nucleotypes and plasmotypes of seven A. thaliana accessions. This panel revealed that both the plasmotype and nucleotype-plasmotype interactions contribute substantially to photosynthetic variation. However, an analysis of organellar genetic diversity of 1531 A. thaliana accessions showed that the seven accessions used in this initial cybrid panel captured only a small fraction of the total genetic diversity. Thus, we constructed a novel cybrid panel combining 60 species-representative plasmotypes with four divergent nucleotypes. High-throughput phenotypic in a range of different environmental conditions, revealed that plasmotypes conferring photosynthetic differences are wide-spread within a species. Several plasmotypes and plasmotype-nucleotype combinations show different photosynthetic responses, stressing the need to produce cybrid panels that include broad sampling of plasmotypes and nucleotypes.
An exciting finding was the discovery that one plasmotype enhanced the recovery of photosystem II efficiency (ΦPSII) after a high- to low-light transition, compared to all other plasmotypes. Faster recovery of ΦPSII generally increases crop yields, thus understanding the underlying mechanism of this plasmotype variant may generate novel insights in the regulation of ΦPSII. A combination of a genetic exclusion strategy and in-depth phenotyping was used to identify the causal gene, NAD(P)H Dehydrogenase (NDH) subunit 6. Further analyses showed that the faster recovery of ΦPSII was the result of reduced NDH activity. Unexpectedly, the reduced NDH activity did not increase, but decrease biomass under fluctuating light conditions compared to the control. This demonstrated a previously unknown trade-off between NDH activity and ΦPSII. This suggests that impact on photosynthesis in a given environment, will often also be weighed by trade-offs conveyed by the allelic variant.
Another A. thaliana accessions produced a consisted reduction in ΦPSII as a result of a well-known mutation in the chloroplast PsbA gene. The nucleotype of this accession conferred substantially increased photo-protection, perhaps to compensate for the reduced ΦPSII. Higher photo-protection may thus have evolved as an adaptation mechanism to the PsbA mutation. Understanding the molecular mechanism of this higher photo-protection is important to better understand how photosynthesis and photoprotection are regulated. A biparental mapping population generated from a cross between Ely and the Col-0 reference accession, with regular photo-protection, revealed no less than 15 Quantitative Trait Loci (QTLs) characterizing the variation in photo-protection. None of the genes so far known to be involved in photo-protection map to any of these QTLs. One of the QTLs was caused by allelic variation for the cpFtsY gene. The protein encoded by cpFtsY is needed for the incorporation of light harvesting complexes into the thylakoid membrane, but this function so far had not been associated with a role in photo-protection.
None of the genes we identified to affect photosynthesis phenotypes were previously known to play a major role in photosynthesis. This implies that allelic variation for many more genes than so far are considered are expected to influence photosynthesis. Disentangling cyto-nuclear interactions at a species-wide level revealed that the genetic architecture underlying photosynthetic variation indeed is highly complex. This complex genetic architecture likely prevents photosynthetic improvements by selection on yield. All of these results shows there is a wealth of genetic solutions that nature has come up with for optimal photosynthesis in a range of different environmental conditions, and these are waiting to be exploited.